Serinol nucleic acids (SNAs), a unique class of synthetic nucleic acid analogs, are designed to mimic the structure and function of natural nucleic acids (DNA and RNA). They stand out in the realm of synthetic polymers, known as xeno-nucleic acids (XNAs), due to their distinct properties. With their ability to expand the chemical diversity of nucleic acids, XNAs, including SNAs, hold immense potential in diverse fields such as biotechnology and medicine. Oligonucleotides modified with SNAs hybridize to both DNA and RNA.
Structure and Composition
The backbone structure of SNAs differs from the natural deoxyribose (in DNA) or ribose (in RNA) sugar backbone structure. In SNAs, the backbone contains serinol (2-amino-1,3-propanediol) units. Serinol is a molecule derived from serine, an amino acid, and consists of an amine and an alcohol group attached to a two-carbon chain. This structural modification results in distinct properties of SNAs, including increased stability and binding affinity.

Figure 1: Structures of PNA, SNA, DNA, and RNA. Serinol (2-amino-1,3-propanediol) building blocks are linked via normal phosphodiester linkages giving them a negative charge. SNAs can be used in siRNAs, molecular beacon and ASOs.
Key Features and Advantages
Enhanced Stability: Compared to natural nucleic acids, SNAs are more stable, making them more resistant to enzymatic degradation. This allows for various applications in biological systems.
High Binding Affinity: The altered backbone structure of SNAs can result in more robust and specific binding to complementary nucleic acid sequences, which is helpful for applications in diagnostics and therapeutics, where precise targeting is essential.
Resistance to Nucleases: Synthetic SNAs are not easily recognized and degraded by nucleases, enzymes that typically break down natural nucleic acids. This extends the functional lifespan of SNAs in biological environments.
Applications
Gene Therapy: Oligonucleotides modified with SNAs enable the delivery of therapeutic genes or oligonucleotides into cells. Their stability and binding properties enhance the efficiency and effectiveness of gene delivery.
Diagnostics: In diagnostic assays, SNAs can be employed as probes to detect specific nucleic acid sequences. Their high specificity and resistance to degradation improve the accuracy and reliability of these tests.
Antisense and RNAi Therapies: Well-designed SNA oligonucleotide can selectively bind to mRNA transcripts and modulate gene expression. This application is particularly relevant for developing treatments for genetic diseases and viral infections.
Research Tools: SNAs can serve as valuable tools in molecular biology research, enabling the study of nucleic acid interactions and the development of new biotechnological methods.
Development of SNAs
Ramasamy et al. (1996) and Benhida et al. (1998) synthesized SNA phosphoramidites to incorporate SNAs into oligonucleotides and found that oligonucleotide duplexes containing small numbers of SNAs are less stable than natural duplexes. One single substitution with an SNA reduced melting temperatures by 0.5 to 12 °C depending on the sequence and location of the substitution. Many years later, Kashida et al. (2011) observed that oligonucleotides fully modified with SNAs can hybridize with SNAs, RNA, and DNA in an antiparallel fashion to form relatively stable right-handed helices. Specifically designed SNA oligonucleotides allow to control their chirality, and reversing their sequences inverses their chirality.
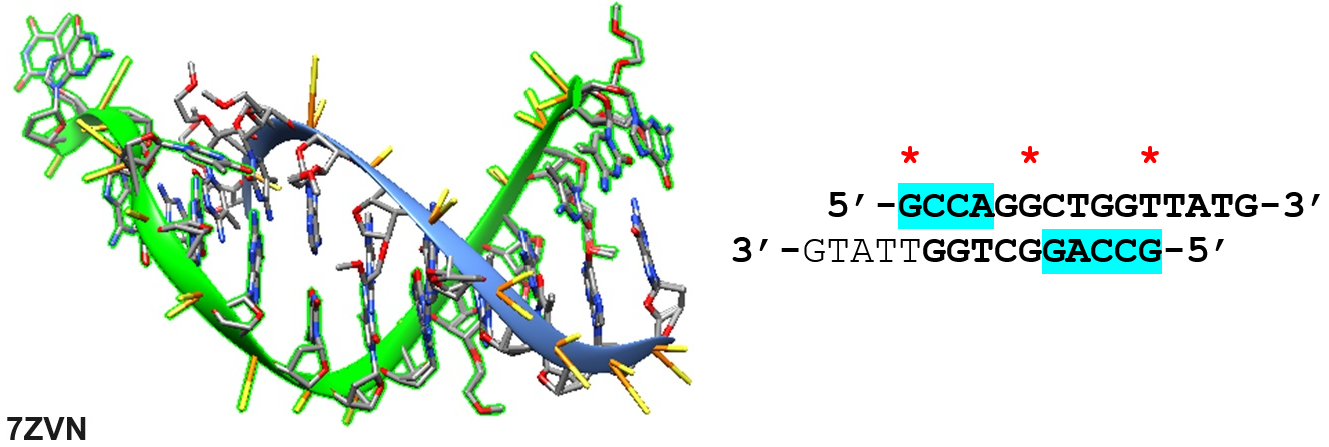
Figure 2: Chemical structures of an SNA phosphoramidite monomer (left). The mirror image of SNA (right) with an asymmetrical sequence ((S)-AT-(R)) is identical to SNA with the reverse sequence ((S)-TA-(R)) (Kashida et al. 2011).
To prevent protein interaction off-target effects of siRNAs, Kamiya et al. (2014) modified siRNAs at terminal ends with SNAs. According to this study, the substitution of SNA into siRNA at both termini of the sense strand and at the 3′ terminus of the antisense strand improved antisense strand selectivity in the formation of RISC, RNAi activity, and resitance to nucleases.
Le et al. (2017) later, reported that SNAs bind RNA more strongly than DNA and showed that SNAs are very stable to nucleases, even to snake venom phosphodiesterase. Le et al. synthesized an SNA antisense oligonucleotide (AO) and investigated its potential to induce exon-skipping, a process where specific exons in a gene's DNA sequence are 'skipped' during gene expression. The study found that the SNA-based AO can effectively induce Dmd exon-23 skipping in vitro. Its related PNA analog showed very high RNA binding affinity but failed to cause significant exon-23 skipping due to poor transfection efficiency with lipid-based transfection reagents.
The phosphodiester linkage between SNA monomers is a key factor that allows for the complexation of SNAs with lipid-based transfection reagents, leading to a superior cellular uptake capability. Moreover, SNA-modified AO demonstrated exceptionally high resistance to nuclease degradation. In 2017, SNA chemistry was a relatively new and unexplored territory for therapeutic applications.
Similar to serinol nucleic acids, Sakata & Fujii (2000) synthesized acyclic adenosine and thymidine analogs containing L- and D-threonine. Using the phosphoramidites of these nucleic acids allowed their incorporation into oligonucleotides using automated solid-phase synthesis. Incorporating threonine nucleic acid analogs into oligonucleotide duplexes did not destabilize hybrid duplexes; however, the analogs' stereochemistry influenced the stability of the resulting duplexes.
Kamiya et al. (2020) solved the crystal structures of RNA hybridizing with SNA and L-αTNA. The heteroduplexes have unwound right-handed helical structures. The structure revealed that base pairs in the heteroduplexes align perpendicularly to the helical axes. The observed unwound helical structures originate from interactions between nucleobases and neighboring backbones of L-aTNA and SNA through CH–O bonds. In addition, SNA and L-αTNA form a triplex structure via C:G*G parallel Hoogsteen interactions with RNA.
 7BPG: Structure of a serinol nucleic acid - RNA complex. |  SNA : RNA Zoom In  SNA building block |
To conclude, serinol nucleic acids represent a promising advancement in synthetic biology. Their unique properties make them suitable for a range of applications in medicine and biotechnology. Their enhanced stability, high binding affinity, and resistance to enzymatic degradation are vital features that differentiate them from natural nucleic acids and other synthetic analogs. As biomedical research progresses, SNAs will likely play an increasingly important role in developing innovative therapeutic and diagnostic solutions.
Reference
Benhida, R., Devys, M., Fourrey, J.L., Lecubin, F., and Sun, J.S.; Incorporation of serinol derived acyclic nucleoside analogues into oligonucleotides: Influence on duplex and triplex formation. Tetrahedron Letters, Volume 39, Issue 34, 1998, 6167-6170. [Science Direct]
Kamiya, Y., Takai, J., Ito, H., Murayama, K., Kashida, H., and Asanuma, H.; Enhancement of Stability and Activity of siRNA by Terminal Substitution with Serinol Nucleic Acid (SNA). Chembiochem, 2014, 15, 2549-55.[onlinelibrary]
Kamiya Y, Satoh T, Kodama A, Suzuki T, Murayama K, Kashida H, Uchiyama S, Kato K, Asanuma H. Intrastrand backbone-nucleobase interactions stabilize unwound right-handed helical structures of heteroduplexes of L-aTNA/RNA and SNA/RNA. Commun Chem. 2020 Nov 6;3(1):156. [PMC]
Kashida H, Murayama K, Toda T, Asanuma H. Control of the chirality and helicity of oligomers of serinol nucleic acid (SNA) by sequence design. Angew Chem Int Ed Engl. 2011 Feb 7;50(6):1285-8. [onlinelibrary]
Kashida H, Nishikawa K, Shi W, Miyagawa T, Yamashita H, Abe M, Asanuma H. A helical amplification system composed of artificial nucleic acids. Chem Sci. 2021 Jan 12;12(5):1656-1660. [PMC]
Le, B. T., Murayama, K., Shabanpoor, F., Asanuma, H., and Veedu, R. N.; Antisense oligonucleotide modified with serinol nucleic acid (SNA) induces exon skipping in mdx myotubes. RSC Adv., 2017, 7, 34049-34052.
Murayama K, Kashida H, Asanuma H. The Use of Serinol Nucleic Acids as Ultrasensitive Molecular Beacons. Methods Mol Biol. 2019;1973:261-279. [PubMed]
Ramasamy, K.S., and Seifert, W.; Amino acid nucleic acids: Synthesis and hybridization properties of a noval class of antisense oligonucleotides. Bioorg Med Chem Lett, 1996, 6, 1799-1804.
Sakata M, Fujii M. Synthesis and properties of novel acyclic nucleotides. Nucleic Acids Symp Ser. 2000;(44):47-8. [PubMed]
SNA-RNA structure: https://www.ncbi.nlm.nih.gov/Structure/pdb/7BPG
---...---